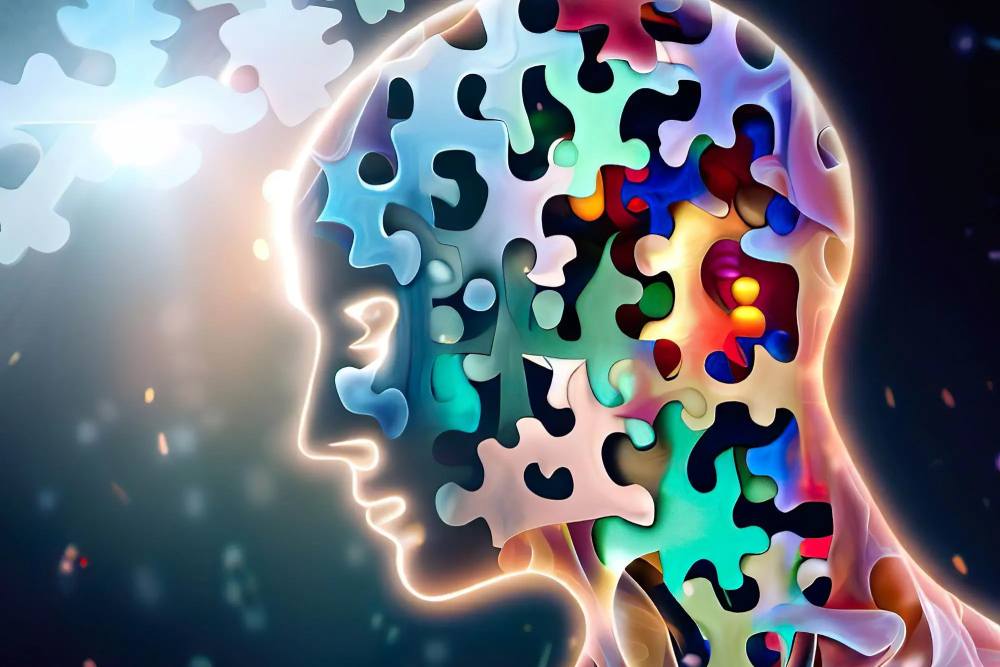
The term “Cytoelectric Coupling” A Breakthrough Hypothesis on How Our Brains Work
- Science
- June 23, 2023
A new hypothesis known as “Cytoelectric Coupling” posits that the physical configuration of the subcellular components of neurons can be changed by the electrical fields generated by neural network activity to improve network stability and efficiency. The study, which was carried out by researchers from MIT, the City University of London, and Johns Hopkins University, builds on previous research that demonstrated how rhythmic electrical activity—also known as “brain waves”—in neural networks and the influence of electric fields at the molecular level can coordinate and adjust the functions of the brain, thereby facilitating flexible cognition.
Information is carried by brain waves. An as of late proposed “Cytoelectric Coupling” speculation recommends that these faltering electric fields add to the streamlining of the cerebrum organization’s effectiveness and heartiness. They do this by impacting the actual arrangement of the cerebrum’s atomic system.
The brain works on many different levels to carry out its many different functions, including thinking. Synchronized electrical activity between neuronal networks depicts information like goals or images. Each neuron’s surrounding proteins and other biochemicals simultaneously carry out the physical mechanics necessary for joining these networks.
The authors of a new study from Johns Hopkins University, MIT, and the City University of London propose a hypothesis they refer to as “Cytoelectric Coupling,” which proposes that the electrical fields of the network have an effect on the physical configuration of the subcellular components of neurons in order to maximize the stability and efficiency of the network.
Earl K. Miller, Picower Professor at MIT and co-author of the paper with Associate Professor Dimitris Pinotsis of MIT and City—University of London, as well as Professor Gene Fridman of Johns Hopkins, stated, “The information the brain is processing has a role in fine-tuning the network down to the molecular level.”
Pinotsis stated, “The brain adapts to a changing world.” Its molecules and proteins also change. They may have electric charges and must catch up to neurons that use electric signals to process, store, and transmit information. It seems necessary to interact with the electric fields of the neurons.”
Thinking in fields Miller’s research focuses a lot on figuring out how the activity of millions of individual neurons can quickly, easily, and still reliably produce higher-level cognitive functions like working memory. By creating and removing connections, known as synapses, as well as by strengthening or weakening those junctions, neurons are able to dynamically form circuits. Miller, however, stated that this merely constitutes a “roadmap” around which information could flow.
Miller discovered that rhythmic activity, more commonly referred to as “brain waves” of various frequencies, coordinates the specific neural circuits that collectively represent one thought.
Fast “gamma” rhythms assist in transmitting images from our vision, such as a muffin, while slower “beta” waves may convey our more in-depth thoughts regarding that image, such as “too many calories.” Miller’s lab has demonstrated that, when timed correctly, bursts of these waves can carry predictions and make it possible to write into, hold onto, and read information from working memory.
They fail when working memory fails as well. A concept known as “Spatial Computing” has been proposed by the lab as evidence that the brain can specifically manipulate rhythms in specific physical locations to further organize neurons for flexible cognition.
Other lab research has shown that, despite the erratic and unreliable participation of individual neurons in networks, the electric fields generated by their collective activity consistently represent the information carried by those networks.
This model of rhythmic electrical activity coordinating neural networks is combined with other lines of evidence that electrical fields can influence neurons at the molecular level in the new study. Cytoelectric coupling
For instance, rather than relying solely on electrochemical exchanges across synapses, researchers have investigated ephaptic coupling, in which neurons influence one another’s electrical properties through proximity of their membranes. This electrical cross-talk can affect how and when neurons in a circuit spike to send electrical signals to other neurons.
Miller, Pinotsis, and Fridman also cite research demonstrating other electrical influences on cells and their components, such as how fields direct neural development and how they can align microtubules.
It is likely that the brain will make use of this capability if the brain transmits information via electric fields that are capable of arranging neurons and other parts of the brain that make up a network. According to the authors, the brain can use fields to make sure the network does what it is supposed to do.
To put it loosely, a television network’s success is more than just its ability to send a clear signal to millions of homes. The minute details, like how each viewer’s house arranges its TV, sound system, and living room furniture to get the most out of the experience, are also important. Miller asserted that the presence of the network inspires each participant to optimally configure their own infrastructure in this metaphor as well as in the brain.
According to the authors of the paper, “Cytoelectric Coupling connects information at the meso and macroscopic level down to the microscopic level of proteins that are the molecular basis of memory.”
The article spreads out the rationale rousing Cytoelectic Coupling. ” Miller stated, “We are providing a hypothesis that can be tested by anyone.”
An example: Coupling cytoelectrically: Dimitris A. Pinotsis, Gene Fridman, and Earl K. Miller, “Electric fields sculpt neural activity and “tune” the brain’s infrastructure,” in Progress in Neurobiology, May 18, 2023.
DOI: The study received funding from the Picower Institute for Learning and Memory, The JPB Foundation, the United Kingdom Research and Innovation (UKRI), and the U.S. Office of Naval Research.